Manticore
RETIRED MOD

- Joined
- Jan 18, 2009
- Messages
- 10,115
- Reaction score
- 114
- Country
- Location
During the wing design process, eighteen parameters must be determined. They are as follows:
1. Wing reference (or planform) area (SW or Sref or S)
2. Number of the wings
3. Vertical position relative to the fuselage (high, mid, or low wing)
4. Horizontal position relative to the fuselage
5. Cross section (or airfoil)
6. Aspect ratio (AR)
7. Taper ratio (
8. Tip chord (Ct)
9. Root chord (Cr)
10. Mean Aerodynamic Chord (MAC or C)
11. Span (b)
12. Twist angle (or washout) (t)
13. Sweep angle (
14. Dihedral angle (
15. Incidence (iw) (or setting angle, set)
16. High lifting devices such as flap
17. Aileron
18. Other wing accessories
http://faculty.dwc.edu/sadraey/Chapter 5. Wing Design.pdf
In aerodynamics, the lift-to-drag ratio, or L/D ratio, is the amount of lift generated by a wing or vehicle, divided by the drag it creates by moving through the air. A higher or more favorable L/D ratio is typically one of the major goals in aircraft design; since a particular aircraft's required lift is set by its weight, delivering that lift with lower drag leads directly to better fuel economy, climb performance, and glide ratio.
The term is calculated for any particular airspeed by measuring the lift generated, then dividing by the drag at that speed. These vary with speed, so the results are typically plotted on a 2D graph. In almost all cases the graph forms a U-shape, due to the two main components of drag.
Lift-to-drag ratios are usually found using a wind tunnel
MACH Aviation Magazine - på webben
In Europe talk was going on, with the German TKF and British EAP as starting points, for a fourth generation fighter, which in due course of time would lead to “Eurofighter 2000/Typhoon”. In France, Dassault was committed to Mirage 2000 and 4000 and was in the very early stage of Rafale-discussions. In Israel, plans for the IAI Lavi, quite similar to Gripen in fact, had set in motion, but later fell prey to the cancellation axe.
Most of these projects had one feature in common, namely the delta canard layout.
At Saab, then in the concept phase of a new fighter, this line of thinking was also the case, which is not surprising. As pioneers of this very aerodynamic shape in the sixties, and with some ten years of Swedish Air Force (RSAF) experience with AJ 37 Viggen at that time, this was quite natural. However, it did not mean that other configurations were neglected.
The Americans obviously intended to stay with the aft-tailed layout, as F-14, F-15, F-16, F-17/18 and F-20 could witness. It had also been reported in the press (AW&ST) that American reconnaissance satellites had caught glimpses of new advanced Soviet fighters on the tarmac of an air base: Ram-J and Ram-L they were called in the CIA jargon and subsequently they became well known as the Su-27 and MiG-29.
The choice of configuration, canard or tail, was far from obvious, initially. A substantial body of knowledge existed on the delta canard layout, gained from Viggen experience of course, but that was not entirely favourable for such a solution.
The close coupled delta canard configuration’s primary feature, its stable vortex flow up to very high angles of attack, meaning high maximum lift coefficient, had lately been realized by the Americans, instead using large strakes as forward wing root extensions together with conventional tail arrangement, as found on the F-16 and F-17/18.
The flow physics are essentially the same. The front surface, being a delta or highly swept strake, gives off a stable detached leading edge vortex that interferes with the vortex flow from the main wing and which mutually reinforces the vortex strength of each other, and therefore burst at a much higher AOA than a lone delta wing would do. This holds true for movements in the pitch plane, but generally not for the other axis, where such flow stability is more difficult to obtain, because of asymmetrical vortex bursting, so modern fighter aircraft generally “stall” first in the lateral and directional axis.
Still the canard layout offered much, if only the weak spots could be cured. First of all a movable canard surface, higher aspect ratio wing and good cross sectional area-ruling and high slenderness ratio had to be incorporated.
Canard layout features
Engine air intake location is a topic of heated debate among aircraft designers. There are usually several options at the early design stage and pros and cons are easy to list for various arrangements. Many air inlet types were contemplated and some underwent both wind tunnel investigations and thorough studies at the drawing boards.
A fixed pitot type air intake, conventionally placed on both sides of the front fuselage, was till an easy choice, because of its simplicity and favourable cost. But everything considered, this type of intake offered most versatility. The pivots for the canards found a natural bed in this structural area and the aerodynamic carry-over loads from the canards onto the upper sides of the fuselage, acting as lift contributors, are substantial. An additional pylon location on the underside of the right intake was another bonus. This is primarily a station for various sensor pods of light weight. The left bottom side is partly occupied by the internal cannon (only for the single seater).
The aerodynamic advantages derived from the close coupled canard configuration, foremost its good vortex flow stability up to high angles of attack (AOA), that can be translated into a very high instantaneous turn rate, and which in conjunction with pivoting canards that are automatically trimmed to give optimal lift-to-drag (L/D) ratios for all cg positions, Mach and AOA, were not technically feasible for the Viggen generation of fighters. Only full span slotted flaps on the canards were present on the Viggen, for further improvement of its already excellent Short Take Off and Landing (STOL) characteristics).
One decisive feature in obtaining good, straight pitching moment characteristics from the type of plan-form was found to lie in the slightly aft sweep of the canard pivot. This was derived through an intensive wind tunnel effort that consisted of testing a formidable number of systematically differing plan-form shapes, both for the main wing and the front surfaces.
In order to successfully meet the often contradictory performance requirements stipulated by the RSAF, a good balance had to be struck between the important wing geometrical parameters, such as sweep angle, thickness, aspect ratio, twist, camber and area.
For example, a demand for high supersonic speed capability and/or low transonic buffeting levels during heavy g-loading will be eased by high wing sweep angle, but then range and manoeuvrability will be degraded accordingly. And a thin wing, good for high speed, might be a blow to rolling performance at high dynamic pressures.
The plan-form that eventually emerged was a good balance between zero-lift, wave, and induced drag and showing a maximum L/D of 9, some 25 percent and 60 percent higher than the previous Saab fighters, the Viggen and Draken respectively. Leading edge sweep angle, actually three different angles for the main wing, is higher on the canard surface to ensure stable flow, as the up-wash there can increase the local AOA substantially.
High angle of attack
The topic of air combat at high angles of attack has gained much interest since the seventies, when it made reappearance, perhaps helped by the not-so-reliable air-to-air missiles of that era. Air combat seemed to end up like a classic dog-fight, with decreasing speed and subsequent high AOA. Many early supersonic fighters had a tendency to stall out of the sky when entering this region of the flight envelope, to the dismay of its pilots, as recovery was often difficult, if not impossible.
The Viggen aircraft had gone through a program of spin testing in the late seventies, that verified the rather benign high AOA characteristics of the canard layout, a fact contrary to what was known on some contemporary aft-tailed foreign fighters. So this was also an argument favouring the Gripen canard layout. Early investigations in vertical spin tunnels and tests in different rotary rigs and subsequent simulations, also pointed to acceptable spin behaviour.
A very substantial flight test program that recently was concluded for both the single as well as the two seat Gripen versions has also fully verified the excellent recovery capability, both in manual test mode and in the normal automatic mode. There exists a requirement in the Gripen project specification for a spin recovery capability, and if this can not be shown, a spin prevention system must not allow a departure to happen. Flight testing has also verified that the EFCS matches this additional demand. Double insurance might be said to exist.
As remarked previously, the only externally visible “fix” to the airframe are a pair of small strakes behind the canard surfaces. This type of “flow augmentation system”, often serving the purpose of directional and lateral stability enhancement at high AOA, is not uncommon on fighters; suffice to mention the Eurofighter and the Mirage 2000.
A spectacular Gripen aircraft departure and ensuing crash at a public air display in 1993, was the cause of modifications and revisions to the EFCS control laws in order to cure certain ailments there, one example being pilot induced oscillations (PIO). Among the changes was one pertaining to canard deflection angles at high AOA in combat mode, to increase margins for the trailing edges surfaces to run into a geometrical limitation, and thus possible longitudinal stability loss and eventual departure.
Yaw and roll stability at high AOA is strongly dependent on canard incidence, and slightly above the MLL boundary, stability drops off rapidly, becoming unstable earlier for canard deflections in the region of minus 10 to minus 25 degrees. Obviously, this incidence range was avoided. Instead small positive values of canard deflection were used in the control law’s schedules. This was beneficial, as it meant that the trailing edge surfaces were positive, that is rear end down, thus giving more positive lift. But now it was realized that in some conditions, a physical, geometrical limitation to the elevons might be encountered, which momentarily caused loss of stability.
A low speed wind tunnel program had immediately been instigated, and for the first time the large low speed wind tunnel model’s electrical engines, that normally were used only to provide discrete incidence changes to facilitate operations, where now deflected continually during a run.
A bunch of “fixing devices” was tried, and success was instant with several of these. The earlier rapid drop of stability was now completely over-bridged, and the plots showed good, continuous behaviour, indicating at dramatic improvement of the flow characteristics in that a delay of separation occurred to slightly higher alphas. A new canard trim schedule could now be introduced that eliminated the risk of the control surfaces being limited in its travel.
The flow phenomenon, commonly called “dynamic lift”, perhaps more aptly called aerodynamic hysteresis, has been the object of intense interest in some countries for decades, not the least has this been the case in Russia. Its best public known, practical application may well be the awesome aerobatic display performed by test pilot V.G. Pugachev and his “cobra” turn in a Sukhoi Su-27.
When these hysteresis effects manifested themselves during high AOA/spin tests in the specially modified second Gripen prototype, they came as no surprise. Years prior, low speed wind tunnel tests with pitching motion of the model had already demonstrated the presence of marked unsteady flow effects, hysteresis, in the post stall alpha regime. Normal force hysteresis was most evident, but all the other components, except side force, had their share.
In the high AOA and spin tests that has taken place since 1996 and recently concluded successfully, the normal tactic was to initiate the tests with a near vertical climb with speed dropping off to near zero and a rapid increase of AOA up to extreme angles, and the aircraft could then be “parked” at 70 to 80 degrees of alpha. When giving adverse aileron input there, a flat spin with up to a maximum of 90 degrees per second of yaw rotation started and could then be stopped by pro aileron input. Recovery followed, whenever commanded.
A very recent test performed in a specially high AOA equipped twin seat Gripen version has recorded a noticeable increase in maximum normal force coefficient over the static data base value, jumping up to 3.2, nearly doubling the static number 1.8.
Wind tunnel and flight test data correspond reasonably well, but it must still be said that modelling these effects are difficult, so normally in high AOA simulations they are neglected. In the future, their inclusion will hopefully improve simulations of more complex behaviour, like departure entrance.
Aerodynamic summary
The salient points in the Gripen aerodynamics are:
Digital fly-by-wire control system and relaxed, negative static stability in pitch (cg far aft) have made the disposition of the delta canard layout, internal as well as external, much easier, whereby:
Optimal cros sectional area ruling, thus wave drag reduction, has been fully realized.
Main landing gear stowed in fuselage, therefore external stores close to cg, small cg-shift that improves flying qualities.
Wing far forward, enabling long tail cone, meaning base drag and local area distribution favourable, and efficient air brake location on tail cone with small transients when deployed.
The direct fall-out of relaxed static stability are:
· Higher trimmed lift.
· Reduced lift dependent drag.
· Reduced supersonic trim drag.
Delta canard’s inherent good aerodynamics are:
· Stable detached leading edge vortex flow, high maximum lift coefficient.
· Positive trim lift on all lifting surfaces.
· Floating canard offers stable aircraft if EFCS fails.
· Good field performance (take off and landing), enhanced by special aerodynamic breaking mode.
· Battle damage tolerance good, “overlapping” control surfaces.
· Potential for future adaptations, like steep approach, fuselage aiming.
· Low buffeting levels made even better with leading edge flaps.
Spin recovery known to be acceptable for close coupled delta canard (not necessarily so for a long coupled canard configuration):
· Proven spin recovery capability for complete cg and AOR range.
· Nor risk of being trapped in a superstall, control authority exists.
A delta wing as on the Gripen offers a light but strong and stiff structure in conjunction with the use of CFC on the outside skins and main spars, even when the relative thickness of the wing is small.
The question of stiffness is vital, as the single-spar aluminium winged Viggen had shown years before. Not initially meeting the severe requirements on roll rate at high dynamic pressures, more hydraulic cylinders for the moving of the inner trailing edge elevons had been added. And the wings broke.
Early into the Gripen project, the industry discussed the trouble that McDonnell Douglas faced in complying with US Navy roll rate demands for the F/A-18. Aileron reversal had occurred during testing of roll rate at high speed/low altitude. The rather high aspect ratio wing lacked enough stiffness and had to be strengthened, adding weight.
At Saab, an intense cooperative work between the aerodynamicists and the strength department was instituted. Flight mechanics simulations had established the required minimum values for the flex-to-rigid ratio of the rolling-moment-due-to-aileron-deflection-derivative, for meeting the very stringent supersonic roll rate demands. The British Aerospace designed CFC wing was fully up to expectations, as flight tests revealed early, allowing a high rate of roll at the critical Mach/altitude/load factor values stipulated.
A delta wing also offers a fairly large volume for fuel and has in general good static and dynamic aero-servo-elastic stability properties, even with large external stores on the wing weapons pylons.
Careful area-ruling was adhered to during the design phase, and constant improvement suggestions flowed from the aerodynamicists to the airframe design engineers. A particular case of point was the front fuselage that was of circular shape initially, but had to yield to a complicated super-elliptical geometry. Significant gains in wave drag and also high AOA behaviour, were among the pay-offs, but the manufacturing department expressed concern over escalated costs.
Airframe summary
Delta wing, multi spar, carbon fibre composite, offering large fuel volume and low weight.
Strong and stiff wing with good aeroelastic properties. High flex-to-rigid ratios for aerodynamic control derivatives.
Fuselage mounted main landing gear means good external stores capability and small cg-shift, thus easier to meet Flying Qalities requirements.
Optimal cross sectional area distribution and mid winged blended body with low drag.
-------------------------------------------------------------------
1. Wing reference (or planform) area (SW or Sref or S)
2. Number of the wings
3. Vertical position relative to the fuselage (high, mid, or low wing)
4. Horizontal position relative to the fuselage
5. Cross section (or airfoil)
6. Aspect ratio (AR)
7. Taper ratio (

8. Tip chord (Ct)
9. Root chord (Cr)
10. Mean Aerodynamic Chord (MAC or C)
11. Span (b)
12. Twist angle (or washout) (t)
13. Sweep angle (

14. Dihedral angle (

15. Incidence (iw) (or setting angle, set)
16. High lifting devices such as flap
17. Aileron
18. Other wing accessories
http://faculty.dwc.edu/sadraey/Chapter 5. Wing Design.pdf
In aerodynamics, the lift-to-drag ratio, or L/D ratio, is the amount of lift generated by a wing or vehicle, divided by the drag it creates by moving through the air. A higher or more favorable L/D ratio is typically one of the major goals in aircraft design; since a particular aircraft's required lift is set by its weight, delivering that lift with lower drag leads directly to better fuel economy, climb performance, and glide ratio.
The term is calculated for any particular airspeed by measuring the lift generated, then dividing by the drag at that speed. These vary with speed, so the results are typically plotted on a 2D graph. In almost all cases the graph forms a U-shape, due to the two main components of drag.
Lift-to-drag ratios are usually found using a wind tunnel
In practice, the multirole model has met with varying degrees of success over the last two decades. An example of a failure would be the MiG-23 Flogger, which never had the air superiority performance to hold its ground, just like the stillborn naval F-111B. More successful were the F-16 and F/A-18, both born as lightweight transonic dogfighters. Their limitation as bombers lay primarily in limited payload radius performance, low level ride quality for deep penetration, and initially with the F-16, limited tools for precision weapon delivery and defence penetration. The most successful example is without doubt the F-15E/I/S which has proven to be almost as good a bomber as the F-111, and improves on the counter-air lethality of the F-15C.
The now classical air superiority aerodynamic performance model is based on the idea of superior energy manoeuvrability, a concept created by the USAF's John Boyd. In this model, a fighter gains a manoeuvre advantage to fire its weapons by outclimbing, outaccelerating, outturning and outlasting its opponent in a manoeuvring engagement.
Clearly flying high energy supersonic manoeuvres will require both wing design optimisation, and plenty of gas to burn. If the supersonic drag characteristics of the wing are not well matched to this model, more thrust will be required in turn limiting persistence, especially if reheat is required to sustain such manoeuvres. Big fighters like the F-15, the evolved Su-27 and the F-22A (and the stillborn and smaller F-16E/XL) have a major advantage in this style of air combat, since the eyeball range argument for small airframes becomes irrelevent. Speed is life, and gas is speed in this game.
The critical factors for range in fast jets are the fuel fraction (a measure of fuel capacity against weight) and the aircraft's drag. A typical rule of thumb for jets is that 75% of the total drag is parasitic drag and only about 25% is lift induced drag. It follows therefore that a range advantage is held by the aircraft which has the higher fuel fraction and lower parasitic drag, factors such as SFC and cruise Mach number being constant.
The other basic aerodynamic parameter of interest is agility, basic measures of which are the combat thrust to weight ratio, and combat wing loading, both defined for an aircraft weight with a given stores load and 50% of internal fuel. Higher thrust to weight ratio translates into better climb rates, acceleration and given similar wing design, sustained turn rates. Wing loading directly affects climb rate and turning performance.
Measures of Fighter Capability






MACH Aviation Magazine - på webben
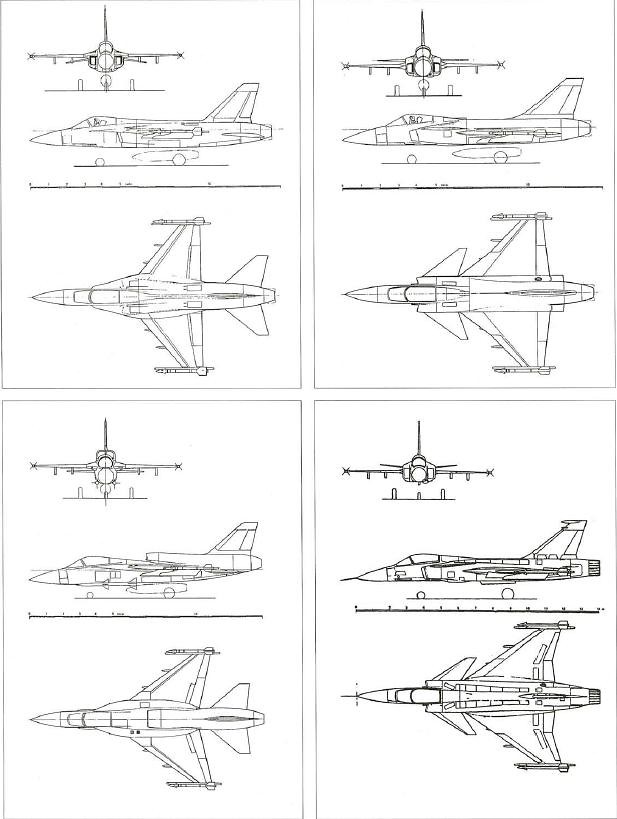
In Europe talk was going on, with the German TKF and British EAP as starting points, for a fourth generation fighter, which in due course of time would lead to “Eurofighter 2000/Typhoon”. In France, Dassault was committed to Mirage 2000 and 4000 and was in the very early stage of Rafale-discussions. In Israel, plans for the IAI Lavi, quite similar to Gripen in fact, had set in motion, but later fell prey to the cancellation axe.
Most of these projects had one feature in common, namely the delta canard layout.
At Saab, then in the concept phase of a new fighter, this line of thinking was also the case, which is not surprising. As pioneers of this very aerodynamic shape in the sixties, and with some ten years of Swedish Air Force (RSAF) experience with AJ 37 Viggen at that time, this was quite natural. However, it did not mean that other configurations were neglected.
The Americans obviously intended to stay with the aft-tailed layout, as F-14, F-15, F-16, F-17/18 and F-20 could witness. It had also been reported in the press (AW&ST) that American reconnaissance satellites had caught glimpses of new advanced Soviet fighters on the tarmac of an air base: Ram-J and Ram-L they were called in the CIA jargon and subsequently they became well known as the Su-27 and MiG-29.
The choice of configuration, canard or tail, was far from obvious, initially. A substantial body of knowledge existed on the delta canard layout, gained from Viggen experience of course, but that was not entirely favourable for such a solution.
The close coupled delta canard configuration’s primary feature, its stable vortex flow up to very high angles of attack, meaning high maximum lift coefficient, had lately been realized by the Americans, instead using large strakes as forward wing root extensions together with conventional tail arrangement, as found on the F-16 and F-17/18.
The flow physics are essentially the same. The front surface, being a delta or highly swept strake, gives off a stable detached leading edge vortex that interferes with the vortex flow from the main wing and which mutually reinforces the vortex strength of each other, and therefore burst at a much higher AOA than a lone delta wing would do. This holds true for movements in the pitch plane, but generally not for the other axis, where such flow stability is more difficult to obtain, because of asymmetrical vortex bursting, so modern fighter aircraft generally “stall” first in the lateral and directional axis.
Still the canard layout offered much, if only the weak spots could be cured. First of all a movable canard surface, higher aspect ratio wing and good cross sectional area-ruling and high slenderness ratio had to be incorporated.
Canard layout features
Engine air intake location is a topic of heated debate among aircraft designers. There are usually several options at the early design stage and pros and cons are easy to list for various arrangements. Many air inlet types were contemplated and some underwent both wind tunnel investigations and thorough studies at the drawing boards.
A fixed pitot type air intake, conventionally placed on both sides of the front fuselage, was till an easy choice, because of its simplicity and favourable cost. But everything considered, this type of intake offered most versatility. The pivots for the canards found a natural bed in this structural area and the aerodynamic carry-over loads from the canards onto the upper sides of the fuselage, acting as lift contributors, are substantial. An additional pylon location on the underside of the right intake was another bonus. This is primarily a station for various sensor pods of light weight. The left bottom side is partly occupied by the internal cannon (only for the single seater).
The aerodynamic advantages derived from the close coupled canard configuration, foremost its good vortex flow stability up to high angles of attack (AOA), that can be translated into a very high instantaneous turn rate, and which in conjunction with pivoting canards that are automatically trimmed to give optimal lift-to-drag (L/D) ratios for all cg positions, Mach and AOA, were not technically feasible for the Viggen generation of fighters. Only full span slotted flaps on the canards were present on the Viggen, for further improvement of its already excellent Short Take Off and Landing (STOL) characteristics).
One decisive feature in obtaining good, straight pitching moment characteristics from the type of plan-form was found to lie in the slightly aft sweep of the canard pivot. This was derived through an intensive wind tunnel effort that consisted of testing a formidable number of systematically differing plan-form shapes, both for the main wing and the front surfaces.
In order to successfully meet the often contradictory performance requirements stipulated by the RSAF, a good balance had to be struck between the important wing geometrical parameters, such as sweep angle, thickness, aspect ratio, twist, camber and area.
For example, a demand for high supersonic speed capability and/or low transonic buffeting levels during heavy g-loading will be eased by high wing sweep angle, but then range and manoeuvrability will be degraded accordingly. And a thin wing, good for high speed, might be a blow to rolling performance at high dynamic pressures.
The plan-form that eventually emerged was a good balance between zero-lift, wave, and induced drag and showing a maximum L/D of 9, some 25 percent and 60 percent higher than the previous Saab fighters, the Viggen and Draken respectively. Leading edge sweep angle, actually three different angles for the main wing, is higher on the canard surface to ensure stable flow, as the up-wash there can increase the local AOA substantially.
High angle of attack
The topic of air combat at high angles of attack has gained much interest since the seventies, when it made reappearance, perhaps helped by the not-so-reliable air-to-air missiles of that era. Air combat seemed to end up like a classic dog-fight, with decreasing speed and subsequent high AOA. Many early supersonic fighters had a tendency to stall out of the sky when entering this region of the flight envelope, to the dismay of its pilots, as recovery was often difficult, if not impossible.
The Viggen aircraft had gone through a program of spin testing in the late seventies, that verified the rather benign high AOA characteristics of the canard layout, a fact contrary to what was known on some contemporary aft-tailed foreign fighters. So this was also an argument favouring the Gripen canard layout. Early investigations in vertical spin tunnels and tests in different rotary rigs and subsequent simulations, also pointed to acceptable spin behaviour.
A very substantial flight test program that recently was concluded for both the single as well as the two seat Gripen versions has also fully verified the excellent recovery capability, both in manual test mode and in the normal automatic mode. There exists a requirement in the Gripen project specification for a spin recovery capability, and if this can not be shown, a spin prevention system must not allow a departure to happen. Flight testing has also verified that the EFCS matches this additional demand. Double insurance might be said to exist.
As remarked previously, the only externally visible “fix” to the airframe are a pair of small strakes behind the canard surfaces. This type of “flow augmentation system”, often serving the purpose of directional and lateral stability enhancement at high AOA, is not uncommon on fighters; suffice to mention the Eurofighter and the Mirage 2000.
A spectacular Gripen aircraft departure and ensuing crash at a public air display in 1993, was the cause of modifications and revisions to the EFCS control laws in order to cure certain ailments there, one example being pilot induced oscillations (PIO). Among the changes was one pertaining to canard deflection angles at high AOA in combat mode, to increase margins for the trailing edges surfaces to run into a geometrical limitation, and thus possible longitudinal stability loss and eventual departure.
Yaw and roll stability at high AOA is strongly dependent on canard incidence, and slightly above the MLL boundary, stability drops off rapidly, becoming unstable earlier for canard deflections in the region of minus 10 to minus 25 degrees. Obviously, this incidence range was avoided. Instead small positive values of canard deflection were used in the control law’s schedules. This was beneficial, as it meant that the trailing edge surfaces were positive, that is rear end down, thus giving more positive lift. But now it was realized that in some conditions, a physical, geometrical limitation to the elevons might be encountered, which momentarily caused loss of stability.
A low speed wind tunnel program had immediately been instigated, and for the first time the large low speed wind tunnel model’s electrical engines, that normally were used only to provide discrete incidence changes to facilitate operations, where now deflected continually during a run.
A bunch of “fixing devices” was tried, and success was instant with several of these. The earlier rapid drop of stability was now completely over-bridged, and the plots showed good, continuous behaviour, indicating at dramatic improvement of the flow characteristics in that a delay of separation occurred to slightly higher alphas. A new canard trim schedule could now be introduced that eliminated the risk of the control surfaces being limited in its travel.
The flow phenomenon, commonly called “dynamic lift”, perhaps more aptly called aerodynamic hysteresis, has been the object of intense interest in some countries for decades, not the least has this been the case in Russia. Its best public known, practical application may well be the awesome aerobatic display performed by test pilot V.G. Pugachev and his “cobra” turn in a Sukhoi Su-27.
When these hysteresis effects manifested themselves during high AOA/spin tests in the specially modified second Gripen prototype, they came as no surprise. Years prior, low speed wind tunnel tests with pitching motion of the model had already demonstrated the presence of marked unsteady flow effects, hysteresis, in the post stall alpha regime. Normal force hysteresis was most evident, but all the other components, except side force, had their share.
In the high AOA and spin tests that has taken place since 1996 and recently concluded successfully, the normal tactic was to initiate the tests with a near vertical climb with speed dropping off to near zero and a rapid increase of AOA up to extreme angles, and the aircraft could then be “parked” at 70 to 80 degrees of alpha. When giving adverse aileron input there, a flat spin with up to a maximum of 90 degrees per second of yaw rotation started and could then be stopped by pro aileron input. Recovery followed, whenever commanded.
A very recent test performed in a specially high AOA equipped twin seat Gripen version has recorded a noticeable increase in maximum normal force coefficient over the static data base value, jumping up to 3.2, nearly doubling the static number 1.8.
Wind tunnel and flight test data correspond reasonably well, but it must still be said that modelling these effects are difficult, so normally in high AOA simulations they are neglected. In the future, their inclusion will hopefully improve simulations of more complex behaviour, like departure entrance.
Aerodynamic summary
The salient points in the Gripen aerodynamics are:
Digital fly-by-wire control system and relaxed, negative static stability in pitch (cg far aft) have made the disposition of the delta canard layout, internal as well as external, much easier, whereby:
Optimal cros sectional area ruling, thus wave drag reduction, has been fully realized.
Main landing gear stowed in fuselage, therefore external stores close to cg, small cg-shift that improves flying qualities.
Wing far forward, enabling long tail cone, meaning base drag and local area distribution favourable, and efficient air brake location on tail cone with small transients when deployed.
The direct fall-out of relaxed static stability are:
· Higher trimmed lift.
· Reduced lift dependent drag.
· Reduced supersonic trim drag.
Delta canard’s inherent good aerodynamics are:
· Stable detached leading edge vortex flow, high maximum lift coefficient.
· Positive trim lift on all lifting surfaces.
· Floating canard offers stable aircraft if EFCS fails.
· Good field performance (take off and landing), enhanced by special aerodynamic breaking mode.
· Battle damage tolerance good, “overlapping” control surfaces.
· Potential for future adaptations, like steep approach, fuselage aiming.
· Low buffeting levels made even better with leading edge flaps.
Spin recovery known to be acceptable for close coupled delta canard (not necessarily so for a long coupled canard configuration):
· Proven spin recovery capability for complete cg and AOR range.
· Nor risk of being trapped in a superstall, control authority exists.
A delta wing as on the Gripen offers a light but strong and stiff structure in conjunction with the use of CFC on the outside skins and main spars, even when the relative thickness of the wing is small.
The question of stiffness is vital, as the single-spar aluminium winged Viggen had shown years before. Not initially meeting the severe requirements on roll rate at high dynamic pressures, more hydraulic cylinders for the moving of the inner trailing edge elevons had been added. And the wings broke.
Early into the Gripen project, the industry discussed the trouble that McDonnell Douglas faced in complying with US Navy roll rate demands for the F/A-18. Aileron reversal had occurred during testing of roll rate at high speed/low altitude. The rather high aspect ratio wing lacked enough stiffness and had to be strengthened, adding weight.
At Saab, an intense cooperative work between the aerodynamicists and the strength department was instituted. Flight mechanics simulations had established the required minimum values for the flex-to-rigid ratio of the rolling-moment-due-to-aileron-deflection-derivative, for meeting the very stringent supersonic roll rate demands. The British Aerospace designed CFC wing was fully up to expectations, as flight tests revealed early, allowing a high rate of roll at the critical Mach/altitude/load factor values stipulated.
A delta wing also offers a fairly large volume for fuel and has in general good static and dynamic aero-servo-elastic stability properties, even with large external stores on the wing weapons pylons.
Careful area-ruling was adhered to during the design phase, and constant improvement suggestions flowed from the aerodynamicists to the airframe design engineers. A particular case of point was the front fuselage that was of circular shape initially, but had to yield to a complicated super-elliptical geometry. Significant gains in wave drag and also high AOA behaviour, were among the pay-offs, but the manufacturing department expressed concern over escalated costs.
Airframe summary
Delta wing, multi spar, carbon fibre composite, offering large fuel volume and low weight.
Strong and stiff wing with good aeroelastic properties. High flex-to-rigid ratios for aerodynamic control derivatives.
Fuselage mounted main landing gear means good external stores capability and small cg-shift, thus easier to meet Flying Qalities requirements.
Optimal cross sectional area distribution and mid winged blended body with low drag.
-------------------------------------------------------------------